Neural Prosthesis – An approach towards replacing lost or impaired biological functionality
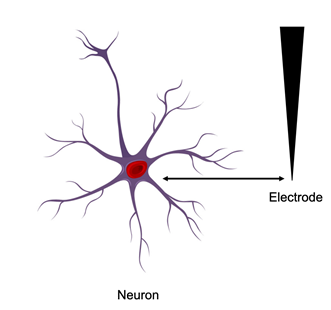
By Bitan Chakraborty, MS and edited by Subha N Sarcar, Ph.D July 12, 2020
Replacing lost or impaired neurological functionalities due to damage or diseases in human is a major challenge in the field of medical sciences. Conventional approaches such as drug therapy, surgery or transplant have been proven to be ineffective in regenerating the biological functionality in such cases and therefore an alternative approach is required.
An approach that has received a lot of attention, especially in the last two decades is an invasive method which requires surgically insertion of an electrode array into regions of the brain, spinal cord or nerve bundles in specific organs. In the United States of America, research efforts in this field is increasing and large amounts of funding are being sponsored by National Institutes of Health (NIH) and Defense Advanced Research Projects Agency (DARPA). Additionally, biomedical companies specializing in the various neuroprosthetics are also investing in an effort to produce functional neural devices.
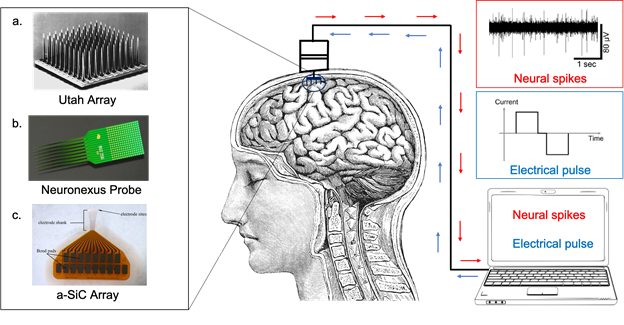
Picture showing bidirectional communication that can be established with the brain using electrode arrays. Example of such arrays (on the left) include a. Utah array (Blackrock Microsystems, LLC.), b. Neuronexus probe (NeuroNexus Technologies, Inc.) and c. a-SiC array made at UTDallas (Neural Interfaces Laboratory). The communication (on the right) is established by recording neural spikes and providing electrical pulses.
Engineering Approach towards Neuroprosthesis
The cardiac pacemaker technology, developed over 50 years ago, was the first implantable electrical interface with the nervous system and since then efforts have been made to translate this technology to control other physiological functions. However, there was only a few clinical and commercial success and some major failures. Therefore, in an effort to improve this technology a more systematic approach has been developed, which has made this field an interdisciplinary one, where scientists, engineers, and doctors would work together towards developing the product, aimed at specific physiological functionality.
In order to successfully make a neural prosthesis the following steps are followed:
- Design and fabrication of the electrode array to be inserted into the nervous system
- Assembly of the array with the electrical interconnect
- Surgically implanting the array into the nervous system
- Establishing a bidirectional communication with the interface and the computer
Each of the steps present their own challenge and requires focus on behalf of the scientist, engineer or doctors to do research in order to come up with solutions. As a result, research institutes and neural prosthetics companies are investing time and money in order to achieve the goal.
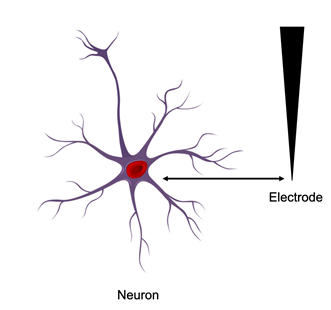
Picture showing an electrode placed in the vicinity of a neuron for the purpose of recording and stimulation.
Neural Recording and Stimulation Electrodes
The electrode arrays interfaces with the nervous system and establishes a bidirectional communication involving sensing of neural spikes (recording) and externally providing electrical pulse to evoke physiological response (stimulation). The array consists of electrode sites capable of recording spikes and providing stimulation and a framework material which provides insulation such that the electrode sites are targeted to specific regions and avoids other regions of the nervous system. Materials used for insulation includes silicon, silicon carbide, diamond-like carbons, and polymers. Electrode materials are generally conducting, where one would think that noble metals like gold, silver, platinum can be used. However, from the safety standpoint, these materials are limited in terms of providing sufficient amount of charge without undergoing reaction with the tissue. Therefore, researchers focused extensively in search of a suitable electrode material, capable of providing sufficient amount of charge safely to evoke physiological response. Current materials used as electrode includes: titanium nitride, iridium oxide, and conducting polymers.
Picture showing an electrode placed in the vicinity of a neuron for the purpose of recording and stimulation.
Once the electrode array is surgically placed near the vicinity of the neurons (either in the brain or the peripheral nervous system), communication must be established to and from the brain, to achieve the prothesis. The activity of neurons is recorded as an extracellular potential (action potential), when the recorded signal identifies the firing of a single neuron. These signals (neural spikes) form the basis of the prostheses by providing cognitive control to the external prosthetic device. Electrical stimulation is applied to depolarize the membranes of the excitable cells in order to initiate the functional response.
Biocompatibility and Safety
A major consideration for developing electrode arrays consists of selecting biocompatible materials. To understand this, test methods have been developed where cells are cultured on these materials, which helps to determine any level of toxic effect of these materials towards cells in contact and during stimulation. Particularly, while providing electrical pulses, caution must be taken in order to avoid electrolysis of water.
Applications of Neural Prosthesis
Neural prothesis is a vast area in terms of research and novel product development. In this area of study, related companies can aim to develop prosthetic devices for specific applications which can be a lucrative investing option for the investors/biomedical companies.
Some of the applications includes the following:
- Upper and lower limb prostheses for spinal cord injury and stroke
- Cochlear and brain-stem auditory prostheses
- Retinal and cortical visual prostheses
- Bladder prostheses
- Cortical recording for cognitive control of assistive devices
- Vagus nerve (Cranial Nerve X) stimulation for epilepsy and depression
- Deep brain stimulation (DBS) for essential tremor, Parkinson’s disease, epilepsy, dystonia, and depression
Outlook towards the Future of this Technology
In spite of the technology being there, this field poses major challenges. One of the challenges is to minimize tissue damage and scar formation due to the implant. Therefore, microelectrode arrays and ultramicroelectrode arrays are being developed so that the dimension of these implants are close to the dimension of the neurons. Another challenge lies in the fact that external cable is required to be connected with the implant, in order to establish the communication with the control computer. A more sophisticated approach needs to be developed such that a wireless communication can be achieved. Extensive research is underway at this point focusing on resolving these challenges in addition to other challenges.
At Neural Interface Laboratory, (one of the bioengineering labs at the University of Texas at Dallas), ultra-microelectrode arrays using amorphous silicon carbide (a-SiC) framework and sputtered iridium oxide electrodes, are being developed and extensive research is underway to understand the effect of design and size on tissue response, life-time analysis of the arrays, and developing electrode material capable of proving high charge safely into the tissue among others.
At this point, the future of this technology seems bright and a successful integration of engineering and medical science is leading the way towards replacing lost/impaired physiological functionality in humans.
References:
Cogan, S. F. (2008). Neural stimulation and recording electrodes. Annu. Rev. Biomed. Eng., 10, 275-309.
Negi, S., Bhandari, R., Rieth, L., & Solzbacher, F. (2010). In vitro comparison of sputtered iridium oxide and platinum-coated neural implantable microelectrode arrays. Biomedical materials, 5(1), 015007.
Kim, S. J., Manyam, S. C., Warren, D. J., & Normann, R. A. (2006). Electrophysiological mapping of cat primary auditory cortex with multielectrode arrays. Annals of biomedical engineering, 34(2), 300-309.
Hetke, J. F., Kipke, D. R., Pellinen, D. S., & Anderson, D. J. (2011). U.S. Patent No. 7,941,202. Washington, DC: U.S. Patent and Trademark Office.
Deku, F., Cohen, Y., Joshi-Imre, A., Kanneganti, A., Gardner, T. J., & Cogan, S. F. (2018). Amorphous silicon carbide ultramicroelectrode arrays for neural stimulation and recording. Journal of neural engineering, 15(1), 016007.
Author: Bitan Chakraborty
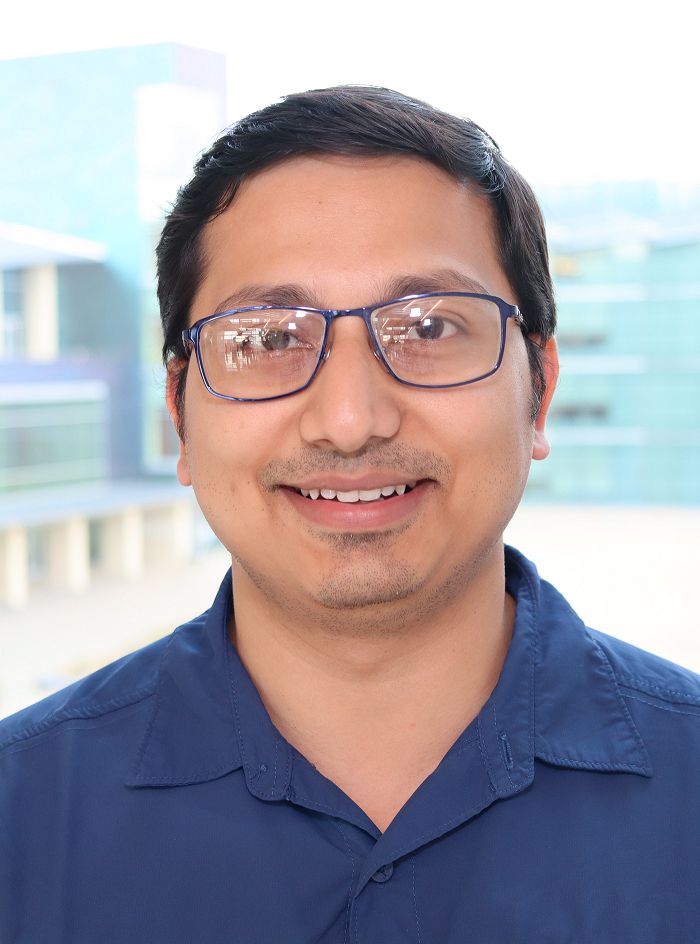